The following text serves as a temporary placeholder, prior to expert moderation
fNIRS
Moderated by: TBD
Functional near-infrared spectroscopy (fNIRS) is an absorption-based, optical technique which makes use of the fact that brain and bone tissue are penetrable by light in the near-infrared (NIR) range, but functionally meaningful chromophores in the brain are not (1–5).
Technical Parameters
fNIRS relies on the injection of light – either directly on the brain tissue or through the scalp – which is sent out to sample the brain. As the NIR-spectrum light attenuation in the scalp, skull and brain tissue is relatively low, the light is able to propagate several centimeters through the brain. As it travels, the light is differently absorbed by the concentrations of oxygenated (HbO), de-oxygenated (HbR), and total hemoglobin (HbT) within the tissue, as they each present with distinct absorption spectra. The corresponding detector is placed several centimeters away and receives a certain amount of diffused light once it has travelled through the tissue (Figure 1). From these changes in optical absorption found in the detected signal, we can reconstruct changes in cerebral hemoglobin concentrations of the tissue below the light source and between the source and detector (1,6).
The exact penetration depth of brain tissue depends on several factors including the source-detector configuration and skull thickness. However, as a rule of thumb the light is not able to travel far enough into the brain to allow for sensitive measurements in deeper cortical regions (1–3,5). fNIRS is able to detect hemodynamic fluctuations in the brain with a spatial resolution in the range of centimeters, which is less than techniques such as fMRI. In terms of temporal resolution, fNIRS is considered to be a fast technique, with sampling frequencies in the range of 100 Hz (millisecond precision) (1–3,5).
Using multiple source-detector combinations (also known as optodes), fNIRS can measure changes in optical properties of the human cortex simultaneously from multiple measurement sites. By exploiting different light-sensitivity patterns of different source–detector distances, so-called tomographic reconstruction of changes in hemoglobin can be achieved. In optical tomography, combining measurements across different source-detector combinations around the scalp (e.g. using a fNIRS cap with a number of electrodes) allows for enough information to be able to reconstruct a slice or a 3D-volume representing the distribution of measured hemoglobin concentrations (3).
Biological Substrate
As fNIRS is able to estimate fluctuations in oxygenated (HbO), de-oxygenated (HbR), and total hemoglobin (HbT), it can provide information on blood dynamics, such as increases in blood volume and flow (1–3,5), which can be associated with neuronal activity, through the principle of neurovascular coupling (NVC). In line with other NVC-based techniques, functional tasks spanning in the range of minutes are often used to evoke hemodynamic fluctiations. So far, results are often presented as 1-D traces of regions of interest (ROIs) or 2D/3D-reconstructions of the cortical surface, see Figure 2 (1). Depending on how much of the tissue of interest needs to be interroagted (e.g. if tomography is attempted), a single acquisiton will also be in the range of minutes.
Unlike BOLD-fMRI, fNIRS provides more information about the evoked-Cerebral Blood Oxygenation (CBO), as it measures the concentration changes of both HbO and HbR (1–3,5). In contrast, BOLD-fMRI measures only the concentration changes of paramagnetic HbR. There is also a difference in the vessel compartiments for which NIRS and BOLD-fMRI are sensitive. NIRS is sensitive to all compartments within the illuminated area: for HbR changes, NIRS is sensitive to the venous and capillary compartments. In contrast, BOLD-fMRI is sensitive to the venous compartment only.
Intra-operative applicability
fNIRS is a particularly cheap, safe and portable with a low susceptibility to motion artefacts (6). In the intra-operative setting so far, fNIRS has been often used in the form of a (hand-held) probe placed directly in contact with the cortical surface (7–9). Outside of the OR, wearable fNIRS acquisition units have already been described (10). One of the major additional benefits of fNIRS in the clinical domain seem to be its lack of signal interference with other techniques, facilitating multimodality approaches. fNIRS has been applied in humans in combinations with for example EEG (1), fMRI (11) and PET (12).
nce with other techniques, facilitating multimodality approaches. fNIRS has been applied in humans in combinations with for example EEG (1), fMRI (11) and PET (12).
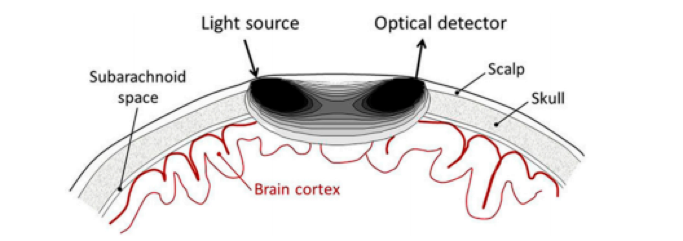

References
- Chiarelli AM, Zappasodi F, Di Pompeo F, Merla A. Simultaneous functional near-infrared spectroscopy and electroencephalography for monitoring of human brain activity and oxygenation: a review. Neurophotonics. 2017;
- Uga M, Saito T, Sano T, Yokota H, Oguro K, Rizki EE, et al. Direct cortical hemodynamic mapping of somatotopy of pig nostril sensation by functional near-infrared cortical imaging (fNCI). Neuroimage. 2014;91:138–45.
- Ferrari M, Quaresima V. A brief review on the history of human functional near-infrared spectroscopy (fNIRS) development and fields of application. Vol. 63, NeuroImage. 2012. p. 921–35.
- Yücel MA, Selb JJ, Huppert TJ, Franceschini MA, Boas DA. Functional Near Infrared Spectroscopy: Enabling routine functional brain imaging. Current Opinion in Biomedical Engineering. 2017.
- Jobsis F. Noninvasive, infrared monitoring of cerebral and myocardial oxygen sufficiency and circulatory parameters. Science (80- ). 1977;
- Boas DA, Elwell CE, Ferrari M, Taga G. Twenty years of functional near-infrared spectroscopy: Introduction for the special issue. NeuroImage. 2014.
- Sato K, Fukuda M, Sato Y, Hiraishi T, Takao T, Fujii Y. Cortico-cortical evoked hemodynamic responses in human language systems using intraoperative near-infrared spectroscopy during direct cortical stimulation. Neurosci Lett. 2016;630:136–40.
- Fukuda M, Takao T, Hiraishi T, Aoki H, Ogura R, Sato Y, et al. Cortico-cortical activity between the primary and supplementary motor cortex: An intraoperative near-infrared spectroscopy study. Surg Neurol Int. 2015;
- Qiu T, Hameed NUF, Peng Y, Wang S, Wu J, Zhou L. Functional near-infrared spectroscopy for intraoperative brain mapping. Neurophotonics. 2019;
- Pinti P, Aichelburg C, Lind F, Power S, Swingler E, Merla A, et al. Using fiberless, wearable fnirs to monitor brain activity in real-world cognitive tasks. J Vis Exp. 2015;
- Kleinschmidt A, Obrig H, Requardt M, Merboldt KD, Dirnagl U, Villringer A, et al. Simultaneous recording of cerebral blood oxygenation changes during human brain activation by magnetic resonance imaging and near-infrared spectroscopy. J Cereb Blood Flow Metab. 1996;
- Hoshi Y, Onoe H, Watanabe Y, Andersson J, Bergström M, Lilja A, et al. Non-synchronous behavior of neuronal activity, oxidative metabolism and blood supply during mental tasks in man. Neurosci Lett. 1994;